Key Takeaway
Pressure control in a switch works through a mechanical or electronic system that senses the pressure level and then activates an electrical contact. In a mechanical pressure switch, a diaphragm or piston pushes against a spring. When the pressure becomes strong enough to compress the spring, it flips a switch — either closing or opening an electrical circuit. This turns a connected device, like a pump or compressor, ON or OFF. You can adjust the spring tension using screws to set your cut-in and cut-out pressures. In electronic switches, a sensor reads the pressure and sends a signal to trigger switching. Pressure switches do not regulate or maintain pressure — they just respond when the level goes too high or low. This makes them ideal for safety systems or basic automation. Their simple control logic and reliability are why they’re used in so many systems — from water tanks to factory lines.
Setpoint-Driven Mechanical Movement
The heart of a pressure switch is its setpoint-driven mechanical movement. As pressure increases, it pushes against a spring-loaded diaphragm or piston. When pressure hits the setpoint, the spring compresses enough to snap the internal contacts—either closing or opening the circuit. This simple mechanism makes pressure switches highly reliable and responsive. Once the pressure drops or rises back across the threshold, the contacts return to their default state. The design may also include hysteresis to prevent rapid toggling. Understanding this internal movement helps in diagnosing problems, such as delayed response or stuck contacts. It’s a purely mechanical logic—compact, durable, and ideal for many real-world applications.
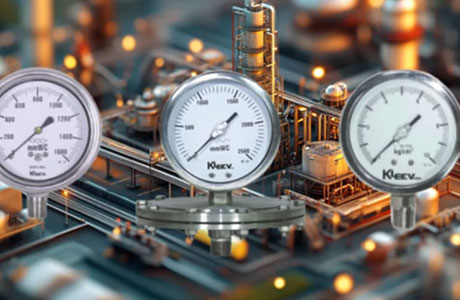
Pressure Sensing Element (Diaphragm/Bellow)
At the heart of the switch is a humble but powerful component: the pressure sensing element. In most industrial pressure switches, this element is either a diaphragm or a bellows.
Now, let’s break it down. Imagine a thin, flexible disc (the diaphragm) or an expandable chamber (the bellows) sitting inside the switch. When pressure is applied, it presses against this element, causing it to flex or expand.
This movement is tiny but crucial. It converts pressure into motion. And that motion is what triggers the next mechanism in the switch—usually a spring-loaded lever or a snap-acting contact.
Why do we use diaphragms or bellows? Because they offer reliability and sensitivity. A diaphragm made from stainless steel or reinforced rubber can last for millions of cycles. In high-precision applications, even a fractional change in pressure can be detected and acted upon.
In fact, I remember a time we installed a pressure switch with a metal diaphragm in a steam boiler system. It had to withstand high heat, moisture, and vibration. After 18 months of continuous operation, it was still spot-on. That’s the level of trust we place in these elements.
You should also know: the choice between diaphragm or bellows depends on the range and type of media. Bellows are great for low-pressure applications with dry gases. Diaphragms are better for liquid systems or where space is tight.
This element is the first responder in your control circuit. If it doesn’t flex correctly, the whole switch fails. That’s why quality and material selection matter.
Activation and Deactivation Logic
Let’s now talk about what actually happens inside the switch when the pressure reaches that magic number.
When the diaphragm or bellows moves enough, it activates a mechanical linkage—often involving a spring, lever, and electrical contacts. This is where electrical control kicks in.
The logic is simple, but the effect is powerful. Once the pressure exceeds the cut-in value, the internal mechanism moves, and the contacts close. That means current can now flow. The switch is ON.
When pressure drops below the cut-out value, the spring force overcomes the pressure force, and the contacts open again. The switch turns OFF. This cycle repeats as pressure rises and falls.
Let me give you an example. In a water pump system, when pressure drops below 30 psi, the pump kicks on. When it reaches 50 psi, the switch opens, and the pump shuts off. This prevents the motor from running continuously, saving energy and extending lifespan.
One key thing you must understand here: the switch doesn’t measure pressure like a transmitter. It reacts to pressure. It’s a binary control device—either ON or OFF.
And it’s fast. Snap-action contacts change state quickly, reducing arcing and wear on the terminals. In control panels, this kind of reliable switching is gold.
So when you see a pressure switch clicking, that’s not just a sound. That’s logic in motion.
Relay Output to External Load
Once the pressure switch activates, what happens next? The electrical output, usually through a relay or direct contacts, controls something downstream.
This is where the pressure switch moves from being a sensor to being a decision-maker.
It might start a motor. Shut down a compressor. Trigger an alarm. Or send a signal to a PLC. The switch output is typically a single-pole, single-throw (SPST) or double-throw (SPDT) relay.
The contacts handle the electrical load. In some cases, they directly carry current to a pump or fan. In others, they just signal a low-voltage control input. Either way, the logic remains the same.
Here’s a real-world story: In our packaging plant, one pressure switch controls an air compressor. The output is wired to the compressor starter circuit. When tank pressure drops, the switch contact closes, and the compressor kicks in. Once pressure builds up, the switch opens, and the compressor shuts off. No PLC. No timer. Just pure pressure-based logic.
And it works. Day in, day out.
That’s why industrial engineers love pressure switches. They offer direct, dependable control with no software bugs, no calibration drift, and no complex wiring. Simplicity wins in tough environments.
Just ensure that the relay contacts are rated for the voltage and current of the load. Overload them, and they’ll burn out.
Impact on System Feedback Loops
Here’s where things get really interesting. Pressure switches don’t just trigger devices—they help balance entire systems.
Every time a switch activates or deactivates, it sends feedback to the system. That feedback is essential to keep everything in sync. Think of it like a thermostat, but for pressure.
In hydraulic and pneumatic systems, pressure switches prevent overload, protect motors, and maintain safe operating ranges. That feedback loop keeps the process efficient and protects equipment.
Take booster pumps, for instance. Without pressure feedback, they might run nonstop, wasting energy and overheating. But with a pressure switch in place, they run only when needed. That’s real-time control.
This kind of feedback is also used in alarms and safety circuits. If the pressure drops too low or spikes too high, the switch can shut down the process or alert the operator. It’s the first line of defense against mechanical failure.
Another example? In a bottling machine, if the air pressure isn’t sufficient, the switch prevents the solenoids from firing. That avoids half-filled bottles and wasted product.
So don’t underestimate the humble pressure switch. It’s not just a trigger—it’s a stabilizer. It helps your automation system breathe naturally.
And because it operates on physics, not code, it’s ultra-reliable.
Conclusion
In most systems, a tiny change in pressure can trigger a major control response. That’s exactly how a pressure switch works. It senses pressure via a diaphragm, bellow, or piston. When the pressure reaches the preset limit, it causes the internal mechanism to snap open or closed—activating or deactivating a connected device. This small movement can start a pump, shut down a compressor, or trigger an alarm. The key to its effectiveness is the switch’s sensitivity and the logic behind activation. Engineers design these switches to respond quickly, reliably, and without hesitation. They also factor in hysteresis—the intentional gap between cut-in and cut-out—to prevent rapid toggling. In feedback loops, pressure switches are essential for maintaining balance and protecting components. Whether it’s a safety cutoff or a start trigger, pressure switches are the gatekeepers of system control. Their subtle actions create big impact, ensuring everything works in sync.